Hydrogen is emerging as a key player in the quest for clean energy. As the most abundant element in the universe, hydrogen has the potential to revolutionize how we store and use energy. However, storing hydrogen efficiently and safely is a significant challenge. This article explores the latest advancements in hydrogen storage technologies, breaking down complex concepts into simple terms.
Why Hydrogen Storage Matters
Hydrogen storage is important for several reasons.
First, it allows us to store energy from renewable sources like wind and solar. This stored energy can be used when the sun isn’t shining or the wind isn’t blowing.
Second, hydrogen can be used in fuel cells to power everything from cars to power plants, producing only water as a byproduct. This makes hydrogen a clean and versatile energy carrier.
Types of Hydrogen Storage Technologies
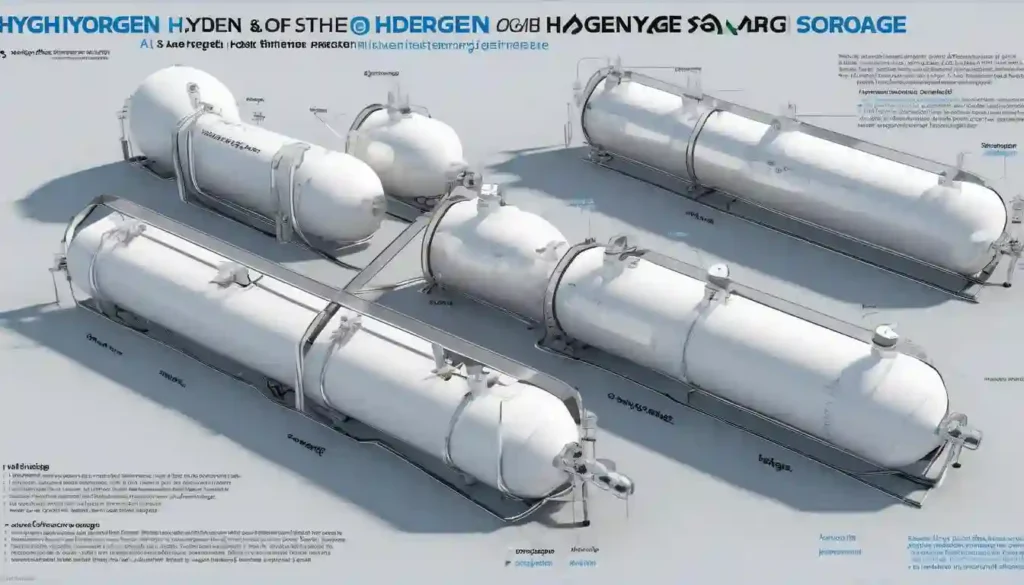
Here are the main types of hydrogen storage technologies:
- Compressed Hydrogen Storage
- Liquid Hydrogen Storage
- Metal Hydride Storage
- Chemical Hydrogen Storage
- Sorbent-based Stora
- Underground or Geologic Storage
Each technology has its advantages and limitations, depending on factors like energy density, storage duration, cost, and application.
Compressed Hydrogen Gas
One of the simplest methods of storing hydrogen is by compressing it into high-pressure tanks. These tanks can store hydrogen at pressures up to 700 bar (about 10,000 psi). While this method is straightforward, it requires strong, heavy tanks and energy to compress the gas.
Liquid Hydrogen Storage
Another method is to cool hydrogen to extremely low temperatures (-253°C or -423°F) to turn it into a liquid. Liquid hydrogen takes up less space than gas, making it more efficient to store. However, the cooling process is energy-intensive, and keeping hydrogen at such low temperatures requires advanced insulation techniques.
Metal Hydrides Storage
Metal hydrides are compounds that can absorb hydrogen gas and release it when needed. These materials can store hydrogen at lower pressures and temperatures compared to compressed or liquid hydrogen. Researchers are exploring various metal hydrides to find the most efficient and cost-effective options.
Chemical Hydrogen Storage
In chemical hydrogen storage, hydrogen is stored in chemical compounds and released through chemical reactions. Examples include ammonia and methanol, which can be easily transported and converted back into hydrogen. This method offers high energy density but requires complex chemical processes to release the hydrogen.
Sorbent-based Storage
- Hydrogen is adsorbed onto materials such as activated carbon or metal-organic frameworks (MOFs).
- Requires low temperatures or moderate pressures.
- Promising for lightweight and compact storage solutions.
Underground or Geologic Storage
- Hydrogen is stored in underground salt caverns or depleted oil/gas fields.
- Ideal for large-scale, long-term storage.
- Suitable for seasonal energy storage and grid balancing.
Emerging Hydrogen Storage Technologies
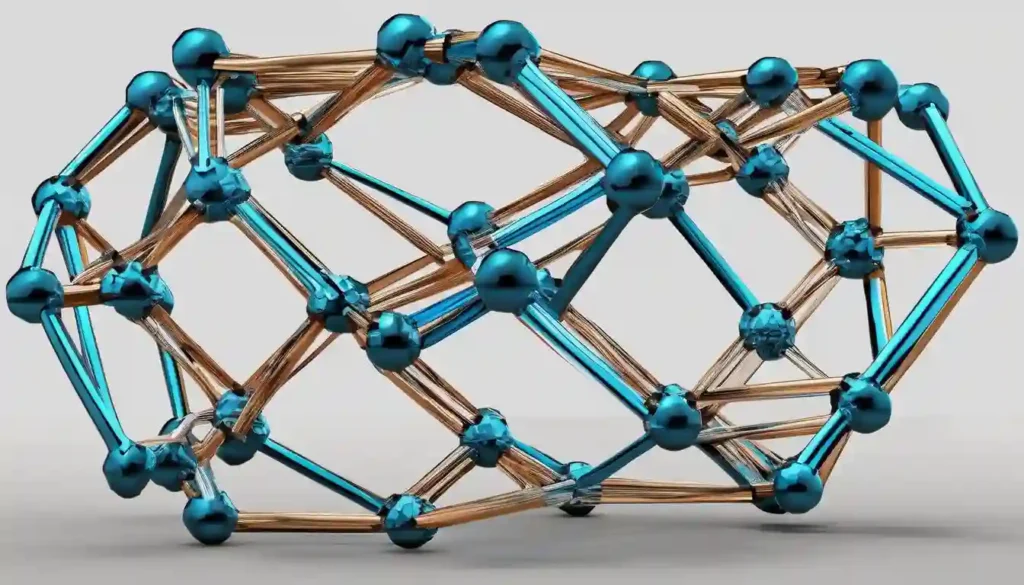
Metal-Organic Frameworks (MOFs)
Metal-organic frameworks (MOFs) are a new class of materials that show great promise for hydrogen storage. MOFs are porous materials made from metal ions and organic molecules. They have a high surface area, allowing them to store large amounts of hydrogen at relatively low pressures. Researchers are working to improve the efficiency and cost-effectiveness of MOFs for practical applications.
Metal-organic frameworks (MOFs) are a fascinating class of materials that have garnered significant attention in recent years. They are known for their unique structure and remarkable properties, making them highly promising for various applications, including hydrogen storage.
What Are MOFs?
MOFs are porous materials composed of metal ions or clusters coordinated to organic molecules called linkers. These components form a three-dimensional network with a high surface area and tunable pore sizes. The metal ions act as nodes, while the organic linkers connect these nodes, creating a cage-like structure.
Structure and Properties
The structure of MOFs can be tailored by selecting different metal ions and organic linkers. This flexibility allows scientists to design MOFs with specific properties suited for various applications. Some key properties of MOFs include:
- High Surface Area: MOFs can have surface areas ranging from 1,000 to 10,000 square meters per gram. This high surface area makes them excellent for gas storage and separation.
- Tunability: The pore sizes and chemical environment within MOFs can be adjusted by changing the metal ions and linkers. This tunability allows for the selective adsorption of different gases.
- Stability: Many MOFs exhibit good thermal and chemical stability, making them suitable for practical applications.
Applications of MOFs
MOFs have a wide range of applications due to their unique properties:
- Gas Storage: MOFs are highly effective at storing gases like hydrogen and carbon dioxide. Their high surface area and tunable pores allow them to adsorb large amounts of gas at relatively low pressures.
- Catalysis: MOFs can serve as catalysts for various chemical reactions. Their porous structure provides a large surface area for catalytic activity, and their tunability allows for the design of specific catalytic sites.
- Gas Separation: MOFs can selectively adsorb certain gases over others, making them useful for gas separation processes. This is particularly important for applications like carbon capture and hydrogen purification.
Challenges and Future Directions of Hydrogen Storage Technologies
While MOFs hold great promise, there are still challenges to overcome:
- Scalability: Producing MOFs on a large scale can be challenging and expensive. Researchers are working on developing cost-effective synthesis methods to make MOFs more commercially viable.
- Stability: Although many MOFs are stable, some can degrade under certain conditions. Improving the stability of MOFs is crucial for their long-term use in practical applications.
- Integration: Integrating MOFs into existing systems and technologies can be complex. Researchers are exploring ways to incorporate MOFs into devices and processes efficiently.
Nanomaterials
Nanomaterials, such as carbon nanotubes and graphene, are being studied for their hydrogen storage capabilities. These materials have unique properties at the nanoscale, allowing them to store hydrogen more efficiently than traditional materials. However, challenges remain in producing these materials at scale and integrating them into storage systems.
Nanomaterials are materials with at least one dimension measuring between 1 and 100 nanometers. To put that into perspective, a nanometer is one billionth of a meter, which is incredibly small. These materials exhibit unique properties that differ significantly from their bulk counterparts, making them highly valuable in various fields.
Types of Nanomaterials
Nanomaterials can be broadly classified into several categories based on their composition and structure:
- Carbon-Based Nanomaterials: These include carbon nanotubes, graphene, and fullerenes. Carbon nanotubes are cylindrical structures with remarkable strength and electrical conductivity. Graphene is a single layer of carbon atoms arranged in a hexagonal lattice, known for its exceptional electrical and thermal conductivity.
- Metal-Based Nanomaterials: These consist of metal nanoparticles like gold, silver, and platinum. They are used in applications ranging from medical imaging to catalysis due to their unique optical and chemical properties.
- Ceramic-Based Nanomaterials: These include materials like titanium dioxide and zinc oxide. They are often used in sunscreens, coatings, and as catalysts.
- Polymeric Nanomaterials: These are made from polymers and can be designed to have specific properties for applications in drug delivery and tissue engineering.
- Composite Nanomaterials: These combine nanoparticles with other materials to enhance their properties. For example, adding carbon nanotubes to polymers can significantly improve their strength and conductivity.
Unique Properties of Nanomaterials
Nanomaterials exhibit several unique properties due to their small size and high surface area:
- Enhanced Strength: Nanomaterials can be much stronger than their bulk counterparts. For example, carbon nanotubes are stronger than steel but much lighter.
- Increased Reactivity: The high surface area of nanomaterials makes them more reactive, which is beneficial for applications like catalysis and chemical reactions.
- Optical Properties: Nanomaterials can exhibit unique optical properties, such as quantum dots that emit different colors based on their size.
- Electrical Conductivity: Some nanomaterials, like graphene, have exceptional electrical conductivity, making them ideal for use in electronics.
Applications of Nanomaterials
Nanomaterials have a wide range of applications across various industries:
- Medicine: Nanomaterials are used in drug delivery systems, imaging, and as antibacterial agents. For example, gold nanoparticles can be used to target cancer cells for imaging and treatment.
- Electronics: Nanomaterials like graphene and carbon nanotubes are used to create smaller, faster, and more efficient electronic devices.
- Energy: Nanomaterials are used in solar cells, batteries, and fuel cells to improve their efficiency and performance.
- Environment: Nanomaterials can be used for water purification, air filtration, and environmental remediation.
- Consumer Products: Nanomaterials are found in everyday products like sunscreens, cosmetics, and clothing to enhance their properties.
Challenges and Future Directions
While nanomaterials hold great promise, there are several challenges to address:
- Safety and Toxicity: The small size of nanomaterials raises concerns about their potential impact on health and the environment. Research is ongoing to understand and mitigate these risks.
- Scalability: Producing nanomaterials on a large scale can be challenging and expensive. Developing cost-effective and scalable production methods is crucial for their widespread adoption.
- Regulation: The unique properties of nanomaterials require new regulatory frameworks to ensure their safe use in various applications.
Solid-State Hydrogen Storage
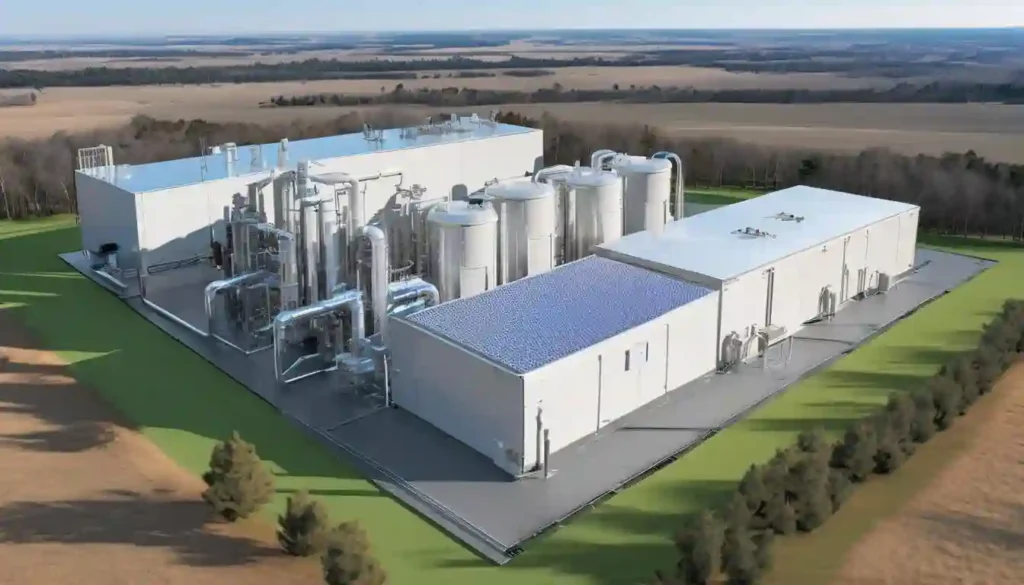
Solid-state hydrogen storage involves storing hydrogen in solid materials, such as metal hydrides or complex hydrides. This method offers high energy density and safety advantages over gas or liquid storage. Researchers are developing new materials and techniques to make solid-state storage more practical and cost-effective.
Hydrogen Storage Challenges and Future Directions
Cost
One of the biggest challenges in hydrogen storage is cost. Producing, storing, and transporting hydrogen can be expensive. Researchers are working to develop cheaper materials and more efficient processes to make hydrogen storage economically viable.
Hydrogen Safety
Hydrogen is highly flammable, and storing it safely is a major concern. Advances in materials and storage technologies aim to improve the safety of hydrogen storage systems. This includes developing better tank materials, leak detection systems, and safety protocols.
Hydrogen Storage Infrastructure
Building the infrastructure for hydrogen storage and distribution is another challenge. This includes developing pipelines, storage facilities, and refueling stations. Governments and private companies are investing in infrastructure projects to support the growing hydrogen economy.
Conclusion
Hydrogen storage technologies are evolving rapidly, offering promising solutions for a clean energy future. From compressed gas and liquid hydrogen to advanced materials like MOFs and nanomaterials, each method has its advantages and challenges. As researchers continue to innovate, hydrogen storage will become more efficient, cost-effective, and safe, paving the way for a sustainable energy landscape.
Keeping an eye on emerging technologies, we can appreciate the potential of hydrogen to transform our energy systems. Whether it’s powering cars, homes, or entire cities, hydrogen storage will play a crucial role in our transition to a cleaner, greener future.